Resonance
noun – PHYSICS. The reinforcement or prolongation of sound by reflection from a surface or by the synchronous vibration of a neighbouring object.
Reverberation
noun – The prolongation of a sound; resonance.
Yes, both are as bad as they appear from an acoustics point of view. These are the two most common phenomenons leading to the hiring of acoustic consultants like Halcyon Audio.
Resonance is pretty much as described above; acoustically, resonance is what we deal with when a material resonates at or close to its natural reverberant frequency. For example, aluminium has a natural resonant frequency of 55.2 Hz, at which it rings or reverberates. This natural resonance is fixed. We can, however, affect and effect this by working, shaping, alloying or otherwise changing the structure of the material. This holds true for all materials. There are no materials that do not have a natural resonant frequency, although some materials can display different natural resonant frequencies at which they are sensitive depending on factors such as shape and hardness.
Reverberation is prolonging the resonant effect of the frequency by energy transmission, either mechanically, electrically or acoustically. The effect can be audible or inaudible anywhere on the frequency spectrum.
As you can see, resonance and reverberation are closely intertwined. The way that sound behaves in a room can be broken into roughly 4 different frequency ranges:
- The first range is below the frequency that has a wavelength (inverse of the frequency) of twice the longest length of the room. In this range, sound behaves very much like changes in static air pressure.
- Above that first range, until wavelengths are comparable to the dimensions of the room, room resonances dominate. This transition frequency is popularly known as the Schroeder frequency (after Manfred R. Schroeder), or the cross-over frequency. It differentiates the low frequencies which create standing waves within small rooms from the mid and high frequencies.
- The third range is a transition to the fourth zone and extends to approximately 2 octaves.
- In the fourth and last range, sounds behave like rays of light bouncing around the room.
As acousticians, we try to deal with this by converting the audible frequencies to inaudible frequencies by damping, diffusing or reflecting the energy. Remember, energy can neither be created nor destroyed, it can only be converted, stored or released.
A real world example
To illustrate resonance and reverberation, you can perfom a very simple experiment: clap your hands in an empty room with no floor coverings or furniture.
You will clearly hear an echo where the sound can be heard more than once, where the reverberant frequencies are the continuation (and sometimes multiplication) of the initial energy release of the clap. The continuous ringing of the clap is the result of sound waves bouncing around before eventually becoming diffused, reflected or absorbed.
By the time you furnish the room, your clap will create the exact same impulse, but the room’s contents will now absorb, diffuse and reflect differently: you will likely be unable to hear any echo or continuing ringing. It’s still there, it has just been “treated”.
In most cases, from an audio point of view, the two issues of resonance and reverberation go hand in hand. You treat one to deal with the other.
You’ll often hear the phrase RT60 bandied around in acoustics circles. This is defined as “the measure of the time for the sound pressure to reduce by 60 dB after an initial impulse”. We use this as a guide measurement to enable us to deal with the issues caused by reverberation and resonance.
Excessive reverberation causes many issues including:
- lack of intelligibility,
- skewing of audio,
- acoustic artefacts, and
- inability to discern directional information.
Defining the correct RT60 (RT for short) is critical in many applications. For example, in education, the RT of a classroom is mandated to be in the region of 0.7 to 0.9 seconds (BB93: acoustic design of schools – performance standards). It has been shown that pupils who are educated in classrooms which meet these requirements absorb and maintain information more quickly and with less error than those who are educated in a less controlled environment.
The desired RT of any space depends on many factors In some cases, we want a reverberant space, like in a concert hall, where we may want a controlled reverberation time of around 1.5 seconds, or more, to give a “live” effect. An anechoic chamber, used for completely different things, needs an RT of zero.
RT is not the same for all frequencies. Ideally, we need to balance the RT across 30 Hz to 12 kHz. It isn’t always possible, of course. But this is what we aim for.
The truth is, we need some reverberation in audio. Without it, places can feel “uncomfortable” and “closed in”. Many people, when experiencing an anechoic chamber for the first time, find themselves panicking because they have no external feedback. A conversation is almost impossible to follow, and, because there is no feedback, there is no spatial awareness. All of these contribute to an unnatural feeling in which panic quickly takes over. An anechoic chamber does not naturally exist in the real world.
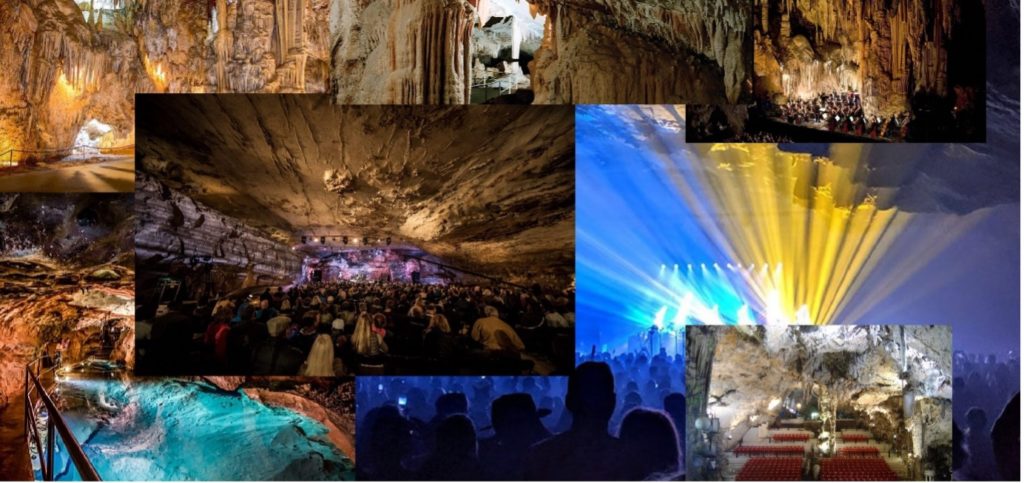
At the other end of the scale, a highly reverberant space, where multiple frequencies are reflected and manipulated, can cause confusion and disorientation leading to paranoia and delusional behaviour. There are some highly reverberant spaces, such as the Caves of Nerja in Spain, St Michaels’ Cave in Gibraltar, Jenolan Caves in Australia and the Cumberland Caverns in the USA, where the natural acoustics make for a wonderful performance space.
Dealing with resonance and reverberation?
We deal with resonance by damping. Damping can be performed on a large scale by using Tuned Mass Dampers (TMD), which must be designed specifically for the purpose and can take hundreds of man-hours to design and test before installation. Small scale damping can be achieved by using opposing magnets held in place to levitate an object, rendering it immune from directly induced resonances.
Reverberation is usually dealt with by converting or storing, and then releasing the kinetic energy in a controlled way, usually using a combination of means most suited to the task at hand, but also across a wide range of frequencies. For example, using a large mass of fibreglass can be very effective for stopping many frequencies from reverberating, through absorption. A curved hard surface can also be used to diffuse and spread those frequencies, instead of reflecting them into the same plane, as you would with a flat surface such as a wall.
Finding the correct balance
There is, unfortunately, no direct or standard method. Unless there is a mandated and specified requirement, there are no rules outside of common sense. We would usually say that for a domestic setting a furnished room should have an RT60 of around 0.8 seconds, or a bit less, which we find optimal in most cases. Bathrooms sometimes have an RT60 as high as 1 second because of the hard surfaces present. Small rooms suffer less reverberation but present more acoustic problems as the energy transfer process is less efficient below 200 Hz.
In an office environment, it would usually be desirable to aim for an RT60 of around 0.6 seconds in a cubicle style environment, and of less than 1 s in a larger open plan style space, before desks and any dividers are installed.
Causes
As we said, everything has a resonant frequency. These can be affected by many factors, not least of which is the natural resonant frequency of any material.
Let’s look at a domestic living room…
You are likely to have a plastered ceiling and walls, both of which will react differently to sound waves as they are built using the same materials in a different way. You are likely to have an amount of glass and some doors as well as some type of floor covering, often laminate hard floor, tiles or carpet.
In such an environment, the only way to get an idea of the overall resonant frequency is to take some basic measurements. They will lead to the calculation of the resonant frequencies which are being produced and will display the effects the room shape, size have. This is how we encounter room nodes. They are caused by sound waves reflecting off various surfaces. They can be determined by the size of the room. The modal response is the biggest determining factor of how a room ultimately “sounds”.
Types of room nodes
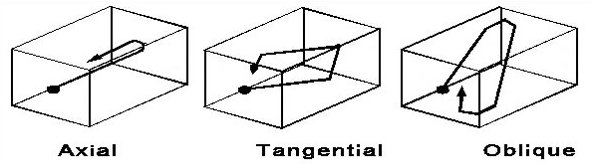
There are 3 types of nodes. Axial nodes are the strongest and the ones we are mostly concerned with. However, both tangential and oblique nodes cause issues, especially where we see several of these grouped together.
Smaller rooms with hard surfaces suffer strong modal responses in comparison to larger rooms, as the fundamental frequency response of a large room is much lower making its tonality more consistent and natural.
A bigger room, however, is not always better!
A good architect will quickly check any given room size to see if it has acoustics issues before committing drawings to the build. It is important to note that the room information only relates to basic modal information, it will not relate to soundproofing, tonality, reverberation or any other acoustic issues. That is the job of a specialist (such as Halcyon Audio).
Application
Let’s look at a real room and “plug” its parameters into a room calculator. This will give us an idea of what to expect. This is what my lounge measurement looks like from an acoustic point of view:
Computed Information:
Room Dimensions: 4.62 m x 4.05 m x3.08 m (L x W x H)
Room Ratio: 1 : 1.31 : 1.5 (L : W : H)
Schroeder Fc: 113 Hz
Frequency ranges:
– No modal boost: 1 Hz to 37 Hz
– Room modes dominate: 37 Hz to 113 Hz
– Diffraction and diffusion dominate: 113 Hz to 452 Hz
– Specular reflections and ray acoustics prevail: 452 Hz to 20,000 Hz
Count (37.2-202hz) : Axials=12, Tangentials=47, Obliques=60
Count (37.2-100hz) : Axials=5, Tangentials=6, Obliques=1
Critical Distance (direct = reverberant field): 4.40 m
Starting with the room’s dimensions, the software gives me room ratios (none of is divisible by any other) and tells me where the dominant room modes are – in this case, 37 Hz to 452 Hz – and that I’m going to see some specular effects (acoustic artefacts) from 452 Hz up to 20 kHz. It also tells me the number of modes in the room and the critical distance of the direct reverberant field, which sits within the length of the room at 4.4 m. That means that I should avoid siting any exciter (speaker) at exactly 4.4 m from the front and rear walls. It also gives me a mean resonant frequency of the room at 113 Hz, with a low frequency mean response of 42 Hz. I can also simulate rotating the room and evaluate the resulting data.

Alongside the above information , I get a Bonello curve alongside a visual layout of the nodes in the room, with a piano keyboard as illustration. I can see that I may have issues between lower C# and D# (three semitones).
It also tells me how the total absorption value (in Sabin) needed for my room, as well as the ratios of diffusion, reflection and absorption. I have an old fireplace in this room; it creates alcoves at its sides which were not considered when establishing the room’s dimensions. Ignoring those alcoves would be a flaw. I know how to deal with them and I can individually work out their acoustical properties. I can use them or discard them depending on how their impact.
If this sounds like too much information for you, then it probably is. But to me, as someone trained in how to interpret these results, it’s really good information to formulate the basis of being able to offer a solution toward acoustics issues presented by any room. The software gives me the correct amounts of diffusion, reflection and absorption I need to apply to deal with resonance, reverberation and tonality.
I now know, for example, that if I was creating a control room in here, I could use the alcoves to soffit mount large monitors, without affecting the modality of the room. I could create an ITU certified room to be tonally even throughout the range with only fairly minor works.
I also hasten to add that these are mathematically known figures, I can work it out without the software, using only a pen and paper. It just takes a lot longer.
Applying it to your room
Very quick calculations, starting from basic data, I can get a good idea of your room’s acoustic properties. I can then tell you what any treatment could look like, if at all necessary very few rooms really need any.
I can tell where you are likely to have problems, and, using other known factors – such as the reverberation index for a plastered wall on a wooden frame or an average sofa – I can get an idea of how much diffusion, reflection and absorbancy are already in the room. I can then work that back to a tonally correct room that sounds natural, with a comfortable level of reverberation and minimal resonance.
Calibrated microphones and measurement software
Software can only do so much. Whipping out a calibrated microphone and taking measurements really should be done last, after evaluating a room’s acoustic properties in the more traditional way. It is a good practice that helps with confirmation and corellation of findings, on top of being a great way of illustrating a report. Finally, it offers a good level of proof that the remediation work performed has reached its objectives.
When I step into a clients space, I will already have had reasonably accurate measurements, which will have allowed me to use the tools already described. I will already know what the client is trying to achieve. I will also already have drawings or photos. I can then use my knowledge, experience, and various tools to come up with the correct solution. The measurement software and microphone will only be confirmation tools, rather than guides.
Software and microphones are powerful tools, leading to powerful potential problems, which we’ll discuss in another article. Chasing a “dream” curve is a futile task.
We appear to have strayed away from resonance and reverberation!
What I hope to have conveyed is that a room is connected to resonances. Those resonances will cause cause reverberations. The scale of those resonances and reverberations is intimately linked to the dimensions and shape of the room.
The room is the most important part of your audio experience, no matter whether it is a superclub dancefloor, a concert hall or a regular living room. While an architect is able to ensure that your room meets basic specifications, he will be unable to ensure its complete acoustic performance or fully grasp what it means to you as a music lover. Acoustic consultants will fill that gap.